Research
Find out more about what we love to do...
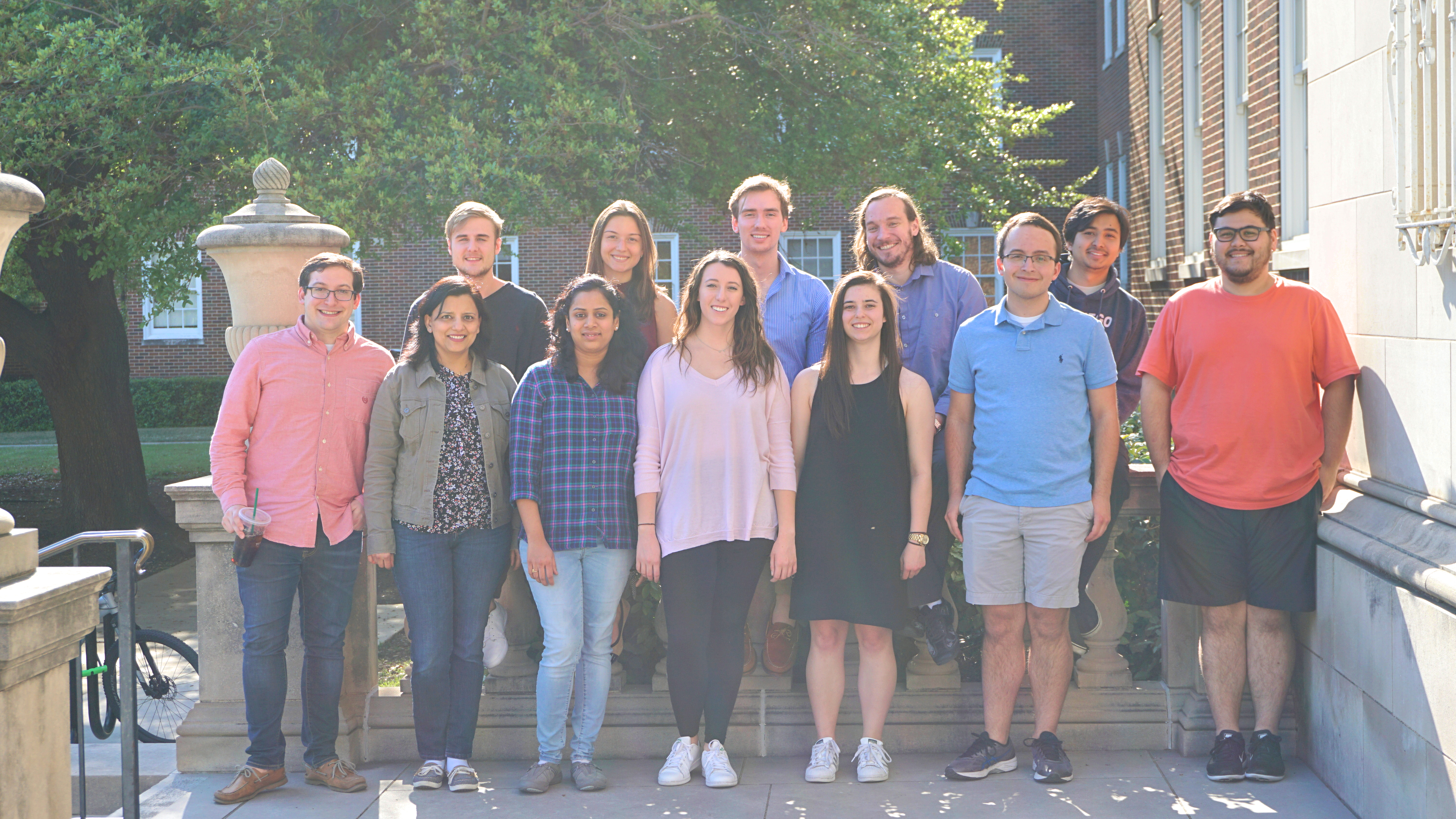
Our primary emphasis is on using biophysical and chemical approaches to understand how organisms adapt to their environment. In principle, we focus on three systems of medical or biotechnological importance: 1) Vertebrate circadian and photoperiodic clocks that are implicated in cancer, diabetes and mood disorders. 2) Plant circadian and photoperiodic clocks that regulate food crop and biomass production. 3) Fungal circadian clocks regulating cellulase production for improved methods of generating biofuels. As a result of this research we are also interested in bioengineering of photoreceptors as optogenetic tools.
Metabolism and the Circadian Clock
Circadian clocks can generically be described as consisting of three elements: Input, an oscillator, and output. In higher organisms input into the circadian clock is primarily through diurnal fluctuations in environmental light and availability of nutrients. In this manner, a master clock within the suprachiasmatic nucleus receives photic stiumli to synchronize peripheral clocks in almost all tissues. Similary, feeding (nutrients) sets a peripheral clock in liver tissue to dictate circadian function. Recent research has identified significant health consequences that result from desynchronization between master (brain) and peripheral clocks. To better understand and regulate these clocks we are using a combination of chemical and biophysical approaches to decipher how metabolic and environemental cues are integrated into circadian clocks to affect both peripheral and master clock fucntion. Our primary focus is on how the protein Cryptochrome mediates crosstalk between complex biological pathways.
Regulation of Plant Growth and Development
We are interested in how circadian and photoperiodic clocks intersect to regulate virtually all aspects of plant growth and development. We are particularly interested in mechanisms of day-length measurement by both blue- and red-light photoreceptors. Current work has focused on looking at chemical mechanisms regulating a class of three homologous Light-Oxygen-Voltage domain containing phtoreceptors, Flavin-Kelch-Fbox 1 (FKF1), Zeitlupe and LOV-Kelch-Protein 2 (LKP2). Despite similar amino-acid sequences and predicted structures, these proteins demonstrate remarkably different photochemistry. How differences in fundamental photochemical mechanisms is related to biological function is currently not well understood. We employ a variety of chemical, biophysical, and computational approaches to examine how these relay variations in environmental blue-light into regulation of biological processes.
Fungal Circadian Clocks and Biofuel Production
Conversion of cellulosic biomass to produce biofuels has been identified as a viable alternative to fossil fuels for our energy needs. One limiting factor in the production of biofuels is the difficult conversion of biomass to fuel sources. Currently, the predominant source of cellulase enzymes for biofuel production is from the fungus, Trichoderma reesei. In T. reesei, cellulase production is regulated by the circadian clock through two blue-light photoreceptors, blue-light receptor 1 (BLR1) and Envoy. Both these proteins harbor LOV domains that are responsible for photic-input into circadian regulation. Currently the mechanisms of Envoy and BLR1 activation are unknown, moreover, how blue-light regulates cellulase gene transcription through activation and repression of the transcription factor BLR1 is unknown. We are interested in understanding the fundamental mechanisms of activation of these proteins to allow improved production of cellulases in T. reesei or genetic engineering of other model fungal systems. We are employing a combination of biochemical and biophysical methods to elucidate molecular models of circadian regualtion of cellulase transcription.
Magnetoreception
Migratory organisms navigate long distances in a seasonal dependent manner. How these organisms are able to navigate long distances with extreme precision is an unanswered question in sensory biology. Currently, it is believed that the protein Cryptochrome, is able to function as a magnetosensor to allow an organism to sense and adapt to the Earth's magnetic field. The chemical, biological, and physical mechanisms allowing this unique function are still not fully understood. Our research group is integrating biophysical, chemical, computational, and biological methods to decipher if and how Cryptochromes are involved in this process. Our goal is to outline a magnetosensor model that links initial photon absorption to behavioral phenomena.
Biotechnology
Photoreceptors are natural, light responsive elements that regulate diverse biological machinery. In the last decade, biophysicists have learned how to harness these proteins to enable light-regulated control over almost any biological process. Engineering of these optogenetic tools has allowed unprecedented abilities to control biology with exquisite spatial and temporal precision. In our group we are particularly interested in the class of Light-Oxygen-Voltage (LOV) domain family of photoreceptors. These blue-light responsive proteins exist as natural plug-n-play modules where a LOV domain imparts light-regulated control of diverse effector domains. Currently, the allosteric mechanisms allowing light-regulation of effector domains is still being deciphered. Our group uses biophysical and computational approaches to decipher new mechanisms that can be engineered to improve the fidelity of LOV-based optogenetic tools..